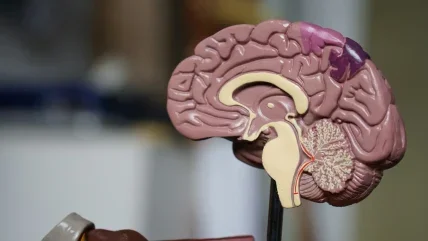
It is no secret that brain surgery is a frightening concept. Surgical interventions are highly effective for reducing or eliminating seizures, but given how highly people value their thinking apparatuses, it should not be a surprise that relatively few patients with drug-resistant epilepsy end up having them.
“As far as I can gauge,” says Kevin Lee, a professor of neuroscience at the University of Virginia, “I think it’s the most underused treatment for a neurological disorder that’s actually highly effective. It’s the hardest thing: I can explain to people that there’s a treatment out there that is going to make them better, but there’s still resistance, even in the medical field, for referrals.
“Once you get people to that point, there’s this reluctance – it’s like saying, ‘Well, do you want to go and have brain surgery?’ There’s a fear of having your skull cracked open – and I get that – and then there are worries about complications.”
It is a bit different with tumours, of course, but that is not because the procedure for removing them is necessarily any smoother. As Shadi Dayeh, an engineering professor at the University of California San Diego, explains, sensors on the manually assembled electrocorticography (ECoG) grids currently used to map brains for resection are spaced 1cm apart, meaning neurosurgeons need to work from an approximate and linear extrapolation of the boundaries between the multifarious wonders and mysteries of their patients’ minds. Although the established practice is to leave wide resection margins – approximately 9mm for language and 5mm for motor function – to minimise the risk of damage, cortical columns in the brain exist on a very different scale from today’s ECoG grids, meaning brain surgeons have to play a sort of dot-to-dot with a knife and the irregular, pulsating tissue inside another human’s skull.
None of this is to denigrate the work of neurosurgeons; their job is idiomatic for a reason. But surely no one would complain if it could be made a little easier. Lee and Dayeh have ways to help with that.
As accurate as possible
Much as they always have been, today’s ECoG devices are made of millimetre-scale electrodes pressed into a layer of biocompatible silicone approximately 1mm thick and then hand-soldered to electrical wires. The difficulty of that process prevents contacts being spaced any less than 1cm apart, meaning grids in clinical use are limited to between four and 64 electrodes. Although larger 16x16cm grids of 256 contacts also exist, their size and expense mean they are only used for research purposes. Not that they would be much more help for a surgery, however big one of these grids gets, its resolution will still be equivalent to a pixel per square centimetre.
That might make it sound too accurate. Tissue is not shaped like computer monitors or TV screens. Thin as a 1mm layer of silicone might seem in those contexts, it is still too thick to fully conform to a human brain, which further complicates things by refusing to stay still.
To address those issues, Dayeh and his team used microfabrication techniques to integrate platinum nanorods into a transparent, biocompatible layer of parylene C. The material choices make all the difference. Parylene C is soft and pliable enough to conform and move with the surface of the brain without obscuring it. “Therefore, you can record with very high fidelity and isolate the activity in local brain regions because the electrical contact is always in intimate contact with the surface of the brain,” Dayeh explains.
Moreover, by fabricating platinum nanorods into the parylene C, the team was able to shrink its ECoG contacts down from the millimetre to the micron scale without introducing extra noise to their readings. “What we wanted to do is make sure we maintained a high capacitance and a facile ability of the electrode to participate in redox reactions,” explains Dayeh. “To do that, you would want to use a highly catalytic material like platinum, but you also need to increase its surface area, so that it’s capacitance is large.” The planar forms used for current ECoG contacts can’t help with that, but structures formed of nanorods are three dimensional. All those nanoscale edges also work to intensify electrical fields, enabling more efficient electrochemical interactions between the brain and the sensor.
“That was the key ingredient for scaling,” Dayeh notes. “Once we accomplished that and validated it with a lower number of channels, we came up with a way to put many of these contacts on grids that are suitable for covering a large brain area.” In fact, the UC San Diego team’s grids, which have been validated in both human and animal models, incorporate 1,024 or 2,048 1mm-spaced ECoG electrodes across a layer just 7μ thick. It is a 100 times increase in resolution.
“We provide a resolution that’s exactly one millimetre spacing, so that you can have basically a resection margin of half a millimetre,” Dayeh explains. “Now you can look at the curvilinear boundary of the tumour, and only resect the tumour and preserve function.”
The precision of PING
Not every neurosurgery needs to be a resection. Advances in using different types of thermal ablation to tackle drug-resistant epilepsy – and even, in some cases, to remove tumours – have made neurosurgery far more accessible and palatable for patients. Kevin Lee would happily speak on the excellences of each different approach, but he is part of a team that has developed a new low-intensity focused ultrasound technique that solves a problem none have yet addressed. He calls it precise intracerebral noninvasive guided surgery, or (in a reference to The Hunt for Red October) PING.
Even the most precise resections and thermal ablations (including high-frequency focused ultrasound) cause collateral damage. It is only the neurons that are responsible for seizures, but all the currently available techniques for removing them also impact nearby blood vessels and axons of passage. “A very common feature of temporal lobe epilepsy surgery is that the more tissue you take out, the better outcome you get,” explains Lee. “The problem is that the temporal lobe, and this holds for the rest of the brain as well, has other axons that are passing to and from it – from areas that are important for other things that you don’t want to damage.
PING is a technique for preventing this sort of damage. Whereas high-frequency focused ultrasound ablates its targets with heat, its low-frequency counterpart just warms them enough that it can be used in conjunction with MRI and an injection of microbubbles to open the blood-brain barrier in a specific area. This makes it possible to inject a neurotoxin (in this case, quinolinic acid), that exclusively damages what Lee calls the “seizurogenic” neurons, leaving glial cells, blood vessels and axons of passage intact. “If you think of the example of surgery in the temporal lobe for epilepsy, those axons of passage would be a quadrant of your visual system that would have been spared,” he explains. “That’s the entire advantage of PING: you hit the culprits that are creating the seizures, but you functionally spare everything else in the area.”
As a result, PING can be used to expand the neurosurgery treatment envelope to areas that are currently too dangerous to treat surgically. Like other focused ultrasound techniques, it uses multiple sonications originating from all around the patient’s head to exclusively target even the most irregularly shaped areas of the brain. “It’s not easy work going in with a scalpel, but with focused ultrasound you can open the blood brain barrier in a specific shape contoured to your target and produce a conformal lesion,” adds Lee, who is quick to highlight what this means for patients. “Resection is a major surgery: you’re going to be in the hospital for a while, you’re going to be in recovery for months; focused ultrasound is pretty close to going to the orthodontist.”
Applying technology the right way
All this can only happen when clinically approved. Lee’s PING procedure and Dayeh’s high-resolution ECoG arrays are still to go through clinical trials. Still, they give an interesting preview of how technology could change neurosurgery in the coming years.
For one thing, Dayeh’s arrays, which also have a very clear use case as brain-machine interfaces, could be used to identify and introduce new clinical markers for epilepsy. Their higher resolution would also make them ideal for directing the use of a procedure like PING on a small subset of aberrant neurons.
Then again, 100 times more information could either make your work 100 times easier or 100 times more difficult. “It’s our job as engineers to make this transition smoother,” says Dayeh. “And the main challenge is how to display and interpret data from 1,000 channels, as opposed to a few tens.”
That is going to require a profound shift from the current practice, which usually involves a neurophysiologist copying ECoG readings of different brain regions from a computer screen onto a printed map. “The surgeon will then try to project based on the context or put a sterile paper on the surface of the brain to mark these regions and match them to the grid,” Dayeh explains. “There’s a lot of back and forth and shouting – and that’s already a big theme in the OR.”
His team is working to smooth the transition with real-time displays of ECoG readings that can zoom in or highlight certain channels to present surgical teams with only the information they need at any given moment in a procedure. “It would really simplify the procedure a lot if we’re able to have a real-time display either directly from the surface of the brain, using light-emitting diodes, or on a computer screen next to the surgeon, where he could visibly see the regions and correlate them with the anatomy on the brain surface.”
As well as supporting better clinical decisions, Dayeh believes high-resolution ECoG sensors combined with real-time displays are a way to shorten and simplify procedures, which would mean lower costs, less risk and greater availability. “This is maybe the holy grail of doing this,” he stresses, “not only to have better assessment and delineation of brain activity, but also to be able to have patients from any background going through the procedure if they need it.”
Lee feels much the same way about PING, which he is confident has the chance to help increase the number of people who take advantage of brain surgery. It could also allow many more people to perform it. Given that they work almost entirely through imaging technologies, it is possible to imagine PING procedures being led by radiologists, who have already begun to play much more central roles in neurosurgery since the introduction of intraoperative MRI.
“Who has his or her hands on the controls of the machine is a good question,” he says. “But you will always need the knowledge base leading up to deciding what the target is. There’ll always be a high-end epileptologist and a neurosurgeon involved in the planning process. And, I mean, I would prefer to have my neurosurgeon at the wheel.” Brain surgery looks like it’s getting a little less complex, but that might just put brain surgeons in even higher demand.
100x
Increase in resolution achieved by UCSD’s next-generation ECoG grids.
93%
Percentage reduction of average seizure frequency in rat models treated with PING.
Experimental Neurology, Volume 343, 113761.