Imagine if you could walk into your doctor’s office and get a complete report on your state of health from just a drop of your blood. You could learn anything from your risk of disease or whether you’re eating too much meat, to which types of treatments may be better suited to you. At least, this is the vision that Dr Luigi Ferrucci, scientific director of the National Institute of Ageing (NIA), is working towards. It would be made possible via proteomics, the study of how proteins function and behave. Doctors would be equipped with technology that identifies all the proteins within a blood sample and collates that data into a unique risk profile for a person. “This is a science that is still young, but it already shows incredible power,” he explains.
Proteomics has only been around since the 1990s, but is billed by some as the future of personalised medicine. This is because proteins can provide exceptionally granular and specific information about what’s happening inside the body. To provide an analogy, our genes provide instructions for how the body should function, while proteins are the workers that carry them out. The problem is that they don’t always do so as planned. Protein activity can change with alterations in the body’s environment, such as with age or as diseases start to develop.
Put another way: genes can indicate what the body might do, but proteins can tell us what it’s doing right now. By studying proteins, we can gain novel insight into the biological changes that precede disease and happen as it worsens.
Not only could this help us discover health problems sooner, but it opens new avenues for drug development and therapy options.
Predicting disease
Because our makeup of proteins can shift as the body enters or approaches a disease state, being able to spot these changes means we can predict illness at an earlier stage. “With pancreatic cancer, when most people go to the doctor with stomach pain it’s too late, it’s stage four,” says Dr Christoph Borchers, professor of oncology at McGill University and director at Segal Cancer Proteomics Centre.
But if we had a routine blood test that could identify a protein that’s a predictive marker for pancreatic cancer, preventative action could be taken. Borchers explains that by looking at someone’s entire set of proteins – known as their proteome – we can look for associations with all kinds of diseases. “This is where we are going,” he adds. “We’re not there today, but it will come very soon.”
Thanks to advances in technology, we can now identify more proteins than ever, but there’s still a way to go. There are thought to be over 20,000 proteins within the human body, but if you got a blood test in the clinic today, it would probably show 30 at most, says Ferrucci.
However, in the lab, tools such as liquid chromatography mass spectrometry can identify upwards of 1,000 proteins, while newer technology, like the SomaScan platform from SomaLogic can spot 7,000 from a 55-μL sample. But as well as identifying a protein, we need to be sure about what it does before making any clinical decisions, says Ferrucci. “There’s a lot of research that’s been done on a large scale – but not as large as I would like to see – to understand the diagnostic power of these proteins.” Eventually, he adds, the aim is to group proteins into profiles that are either associated with, or predictive of, disease.
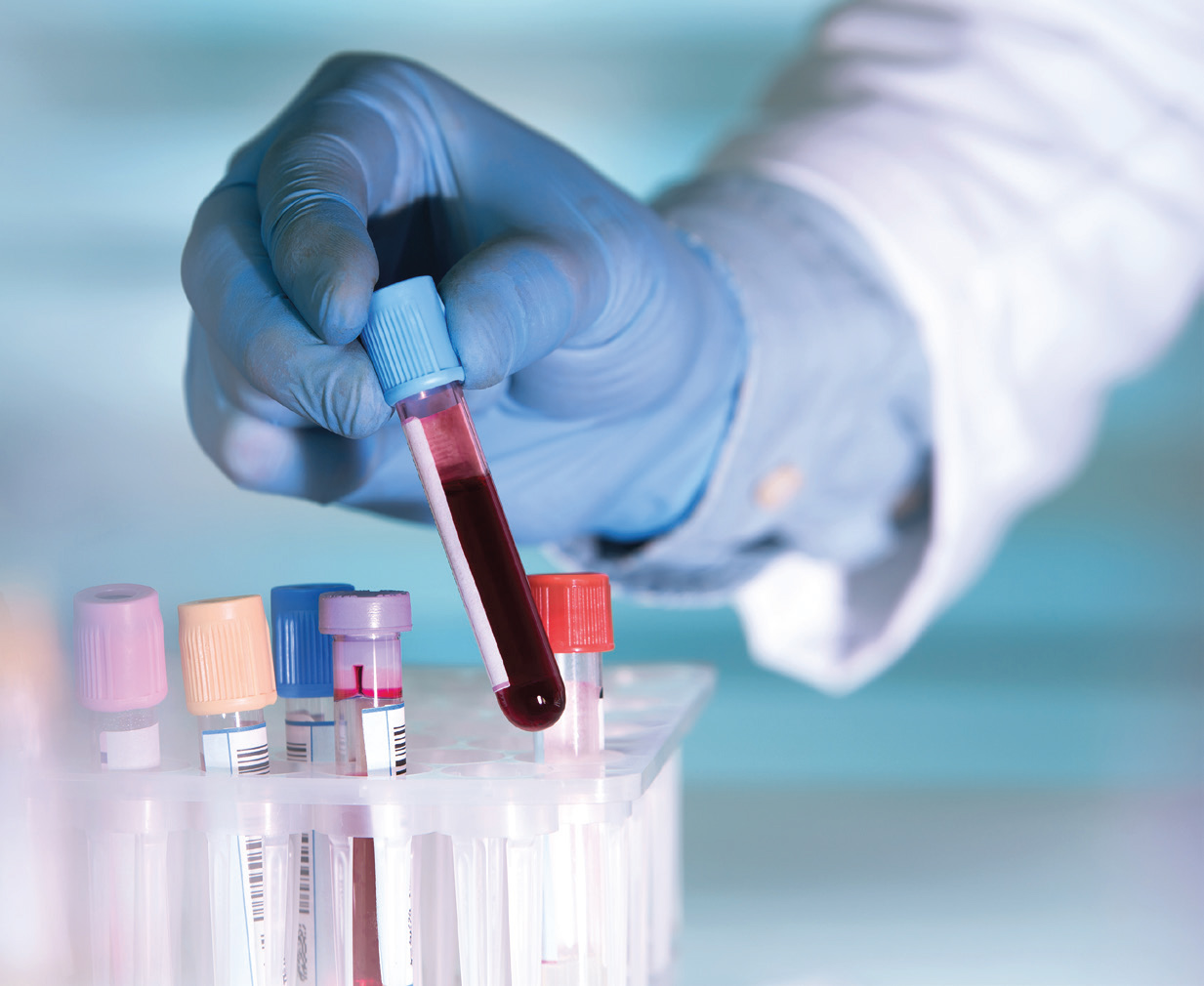
New drug targets
“When we are looking at diseases, we’re not looking at one protein. We’re not even looking at several proteins, we’re looking at pathways now,” says Borchers. Proteins carry out vital jobs, including fighting infection and regulating essential mechanisms. When they behave in different ways this creates biological changes within the body – which can disrupt our functioning and lead to disease developing. If we can identify which proteins play important roles within these pathways, we can target them with drugs that inhibit their activity. “We can say, ‘Look, all of a sudden, this pathway is upregulated. It looks like this protein has a major role. If you knock it out, what happens?’,” Borchers explains.
“When we are looking at diseases, we’re not looking at one protein. We’re not even looking at several proteins, we’re looking at pathways now.” Dr Christoph Borchers
Analysing the shape of the protein, known as structural proteomics, can also give us an insight into how it behaves within a pathway. When a protein changes its shape, this alters its function. Take a neurodegenerative disease that’s brought on when particular proteins change their shape and clump together. If you knew how their structure changed to get to that point, you could create a drug that prevents those changes from happening – which could stop the disease from developing, says Borchers. This may be where we’re headed, but we first need to make sure we can determine which proteins are causally related to disease, says Ferrucci. For instance, a protein may be associated with stress but have a useful function, like showing how your body is reacting to it. So, you wouldn’t want to inhibit that protein. “That won’t be a target, but something causally related to the disease will become a target,” he explains. “At this stage it’s very difficult to understand: what is a biomarker? And what is a causal signal?” To get there, we need more insight into which proteins and their interactions are linked with disease. “You need to study the function of the protein,” says Borchers. “Then you can interfere.”
“We can create drugs that affect the splicing variants generated. This is a formidable pharmacological target for the future.” Dr Luigi Ferrucci
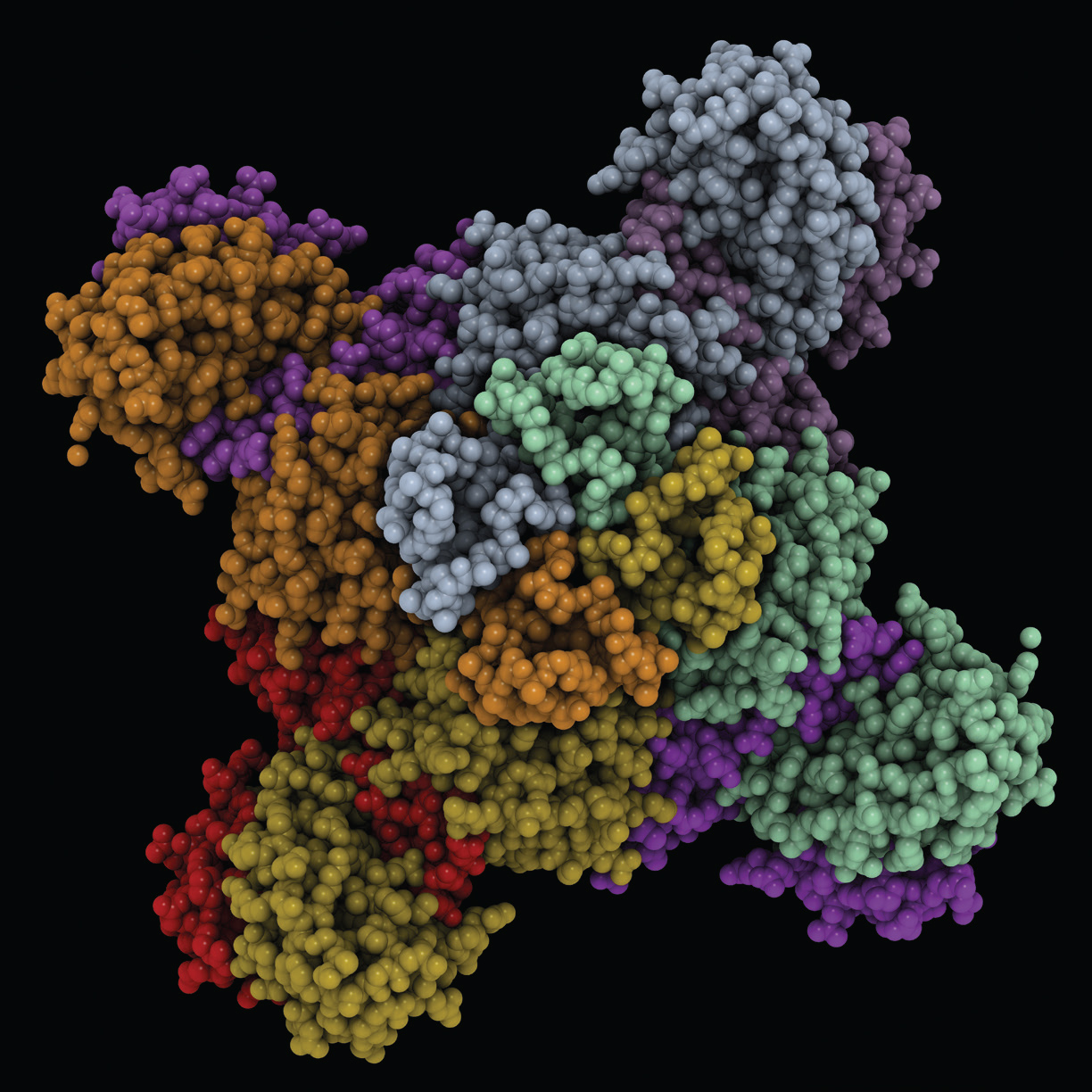
Managing disease
To make matters more complicated, instructions from our DNA can be amended before they’re delivered to proteins, which can generate what’s known as splicing variants. These proteins can influence how a disease develops. Splicing variants happen when a molecule called RNA, which copies and passes on DNA’s instructions, doesn’t copy them perfectly: RNA may add, delete or replace certain bits. A protein is then formed to carry out these altered instructions, but it’s a variation of what would have been made had the instructions been copied correctly. If we can identify splicing variants, we may be able to predict how a disease will affect the body and take steps to manage this, says Ferrucci. “We can create drugs that affect the splicing variants generated. This is a formidable pharmacological target for the future,” he adds.
Ferrucci gives an example: in people with peripheral artery disease, two splicing variants have been identified for VEGF, a protein that promotes the growth of blood vessels. But each variant has an opposite effect: one can improve symptoms by helping blood vessels to form, and the other works against this, making the disease more severe. Targeting the latter variant with a drug could improve the quality of life for millions living with the condition. To find splicing variants, you need to have technology that can read long strands of RNA and figure out where the DNA’s instructions have been altered. “By doing this, people are finding many more splicing variants, but this research is not in the realm of clinical application,” says Ferrucci.
While amended RNA indicates that splicing variants are present, it can’t tell us how many modified proteins are actually generated. One strand of RNA can create tens or even hundreds of proteins. Identifying the number of mutated proteins can help us decide whether to use certain drugs, says Borchers. He shares an example where he and his team investigated the suitability of metastatic colorectal cancer patients for anti-epidermal growth factor receptor drugs – a key strategy in the treatment of the cancer subtype. Having a modification in the KRAS protein disqualified them, and one of the patients had this mutation, but in only 10% of their K-ras proteins – meaning that the remaining 90% could still be targeted by the drug. Had the team looked at genetic data alone, they’d have identified the RNA instructions for creating the modified KRAS protein but not known how many were made from them. They’d simply recognise that this mutation was present, and that patient wouldn’t have qualified for the drug at all. Borchers hopes that soon, it will be standard for proteomics data to inform doctor’s decisions about cancer treatment.
The future of precision medicine
While it may take decades to realise the potential of proteomics, the future looks bright. We already know the biological function of proteins, but we’re onto a winner if we can use them as biological markers of disease. “If we do that, that is the next frontier. In order to do this, people need to work together. Nobody has enough data to make a step forward,” says Ferrucci. Borchers predicts that a person’s protein data will one day be seen as their “blood passport” – a profile of proteins unique to them, like their fingerprint. Doctors could check for any changes to it as part of a regular check-up. In the meantime, we’ll see proteomics technology used by leading labs gradually trickle down to the clinic, he adds. “In the end, science will always win.”